ANU scientists pioneer research for a clean energy revolution
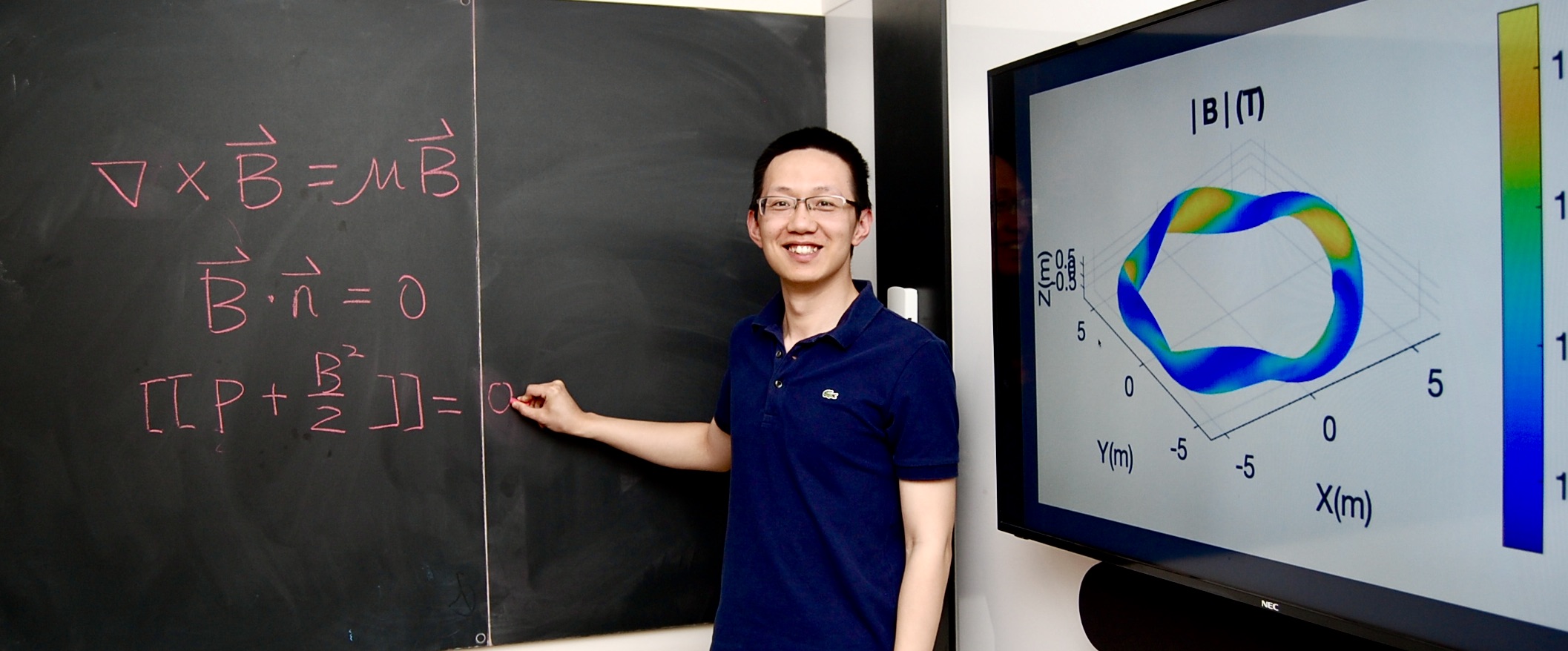
Imagine if the world was able to release its grip on fossil fuels and satisfy its growing appetite for energy, with a clean fuel source and almost no carbon emissions. There’s a developing technology that could transform this vision into reality: fusion energy.
Fusion energy seeks to replicate the reaction that powers our Sun, where two very light atoms are fused together resulting in a substantial release of energy.
The leading concept to realise fusion power is a machine called a tokamak. A tokamak is a doughnut shaped vacuum chamber in which intense magnetic fields are generated to confine a soup of positively charged ions and negatively charged electrons called a plasma. The excellent confinement of a tokamak is achieved thanks to a high degree of (rotational) symmetry and the twisting of magnetic field due to internal currents.
‘The next step fusion energy experiment’, ITER, is a tokamak, and is currently under construction in the South of France. ITER, the world’s largest scientific project, will demonstrate the feasibility of fusion power. Complementary to this experimental technology is another pioneering magnetic confinement device called a stellarator.
In the stellarator, the magnetic field is exclusively produced by coils, that wrap around the plasma in a harmonious but contorted way. The twisting of field-lines, so important for particle confinement, is achieved by deforming, twisting, kinking and bending the doughnut shape. Although the stellarator has slightly poorer particle confinement, it is intrinsically a steady-state (continuous) and less prone to disruption, and hence more suited as a power plant. Because of three-dimensional and asymmetric qualities of a stellarator field, the class of equilibrium solutions is significantly greater than for the tokamak.
In order for atoms to fuse, they must be heated to almost inconceivable temperatures, 150-300 million degrees Celsius. That’s 10-20 times hotter than the core temperature of the sun. To contain this heat, the ionised gas known as plasma, is twisted to prevent it touching the sides of the reactor. The resulting combination of the twisted plasma and extreme temperature, is that the particles no longer collide but fuse, releasing massive amounts of energy.
Dr Zhisong Qu from the Mathematical Sciences Institute (MSI), is among a group of scientists at the ANU and University of Western Australia (UWA), who in collaboration with colleagues at Princeton Plasma Physics Laboratory (PPPL) and the Swiss Plasma Center (SPC), École polytechnique fédérale de Lausanne (EPFL), have accelerated a parallelised supercomputer code by up to a factor of 1000. The code, affectionately referred to as SPEC (Stepped Pressure Equilibrium Code), is a plasma equilibrium code used to construct magnetic field solutions in a stellarator, a doughnut shaped magnetic confinement device designed to harness the power of fusion energy. If it works, fusion power offers vast amounts of clean energy with a near limitless fuel source and virtually zero carbon emissions.
In a recent publication (IOPScience, Plasma Physics and Controlled Fusion, Vol 62, Number 12), Dr Zhisong Qu, Dr David Pfefferlé (UWA) and colleagues, have implemented a new method to compute solutions of the plasma equilibrium problem. The technique is based on the so-called Zernike polynomial representation, which has the huge benefit of having sped the SPEC code by up to a factor of 1000. The result is important as it enables SPEC to be used in a stellarator optimisation code to design a new stellarator. SPEC, which was written by Dr Stuart Hudson of PPPL (and an ANU graduate) is formulated on a Multi-region relaxed MHD theory model pioneered by Em/Prof. Robert Dewar, ANU. This model is the only mathematically rigorous treatment able to capture the full magnetic field complexity of nested flux surfaces, magnetic islands and chaotic fields.
“This research would not be possible elsewhere in the world. It is unique to ANU and our collaborators” explains Zhisong. “From the physics perspective, the model was developed originally by Prof. Robert Dewar and A/Prof. Matthew Hole here at MSI, so we have a strong expertise in understanding the properties of the model itself. In terms of my research in developing the code, I have benefited greatly from the knowledge and support provided by my MSI Computational Mathematics group colleagues in formulating the maths and solving the mathematical problems. I received direct help from members of the group such as Dr Kenneth Duru and Prof. Markus Hegland. Finally, of course, our collaborators from UWA and elsewhere in the world are all very brilliant. It’s truly been a team effort”. We are trying to solve a difficult physics problem, but how we solve this problem is a mathematical challenge” says Zhisong. “To me, maths is the enabling language of Science”.
Having access to the National Computational Infrastructure (NCI) has been crucial to the research of Dr Qu and his collaborators. “It has really accelerated the process. What use to take hours can now be achieved in minutes.” affirms Zhisong. “The NCI supercomputer was heavily used. It is the platform on which we test and run the code. We hope to setup NCI as one of the main supercomputers on which SPEC will be running regularly and to support future research. For this particular research, finding the best possible optimisation can only be accomplished by a high-speed computer because the plasma needs to be twisted. It’s a complicated and highly complex system. The NCI supercomputer is a tool that allows a new class of design to be explored” he explains. “I was relived and happy to see this result from our research. It’s incredibly satisfying and a great feeling of accomplishment”.
Zhisong complete his undergraduate studies at Peking University, Beijing, China. He came to the ANU in 2012 to begin his doctoral studies under the supervision of A/Prof Matthew Hole. Following his graduation, Zhisong spent some time working in industry roles but soon made the transition back to research. “I feel it’s more interesting to be working in science. I’m doing what I always wished”.
A/Prof. Matthew Hole, who leads the Plasma Theory and Modelling Group at the ANU says “This is a game-changer, with the potential to accelerate the entire class of magnetic equilibrium solvers, leading to faster and more accurate solvers”.
The work is funded by the US Simons Foundation, an agency set up by the billionaire mathematician Jim Simons, to advance the frontiers of research in mathematics and the basic sciences.
This web article was published on Thursday 5 November, 2020